
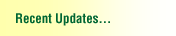
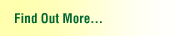


|
We are part of the West Penn Allegheny Health System
|
|

|
Biofilm Research

The Biofilm Paradigm of Infectious Disease

|

|

|
Bacteria in biofilms tend to be more difficult to culture and more resistant to control strategies (antibiotics and biocides) and host defenses than when grown planktonically in the laboratory. Their resilience has been related to physiology and protection by the EPS slime matrix that they produce. These phenomena may explain seemingly conflicting features of the disease when signs and symptoms are otherwise consistent with infection.
- Chronic in Nature
- Culture Negative
- Poor Response to Antibiotics
- Potential for Metastasis
|

|
|
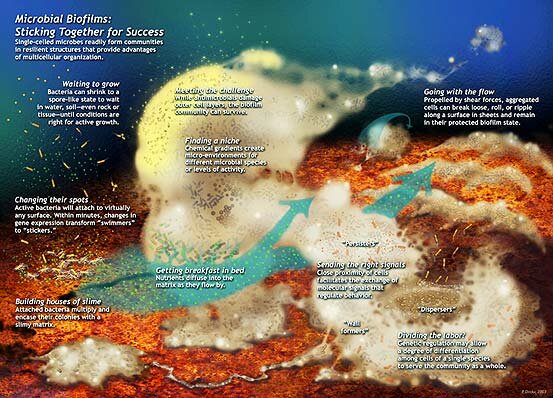
The more we study biofilms the more we found out about their structural complexity and development. This schematic was drawn by Peg Dirckx from the Center for Biofilm Engineering to incorporate various biofilm behaviors and concepts based largely on observations from confocal and time-lapse microscopy. An interactive version can be found at http://www.erc.montana.edu/MultiCellStrat/default.html. A version of this schematic has also been published in Nature Reviews Microbiology 2, 95-108 (2004). Click on the image for a larger version.
Confocal image (left) of a Mycobacterium fortuitum biofilm illustrating key biofilm characteristics. The individual bacterial cells are stained green with a nucleic acid stain which stains the DNA. The surrounding EPS matrix is stained red with a lipophilic stain, giving both locational and compositional information. The cells and EPS are organized into a secondary structure of cell clusters separated by water channels and voids (black areas). M. fortuitum is an opportunistic bacterium that inhabits water and can form biofilms. It can cause cervical lymphadenitis and otomastoiditis in children as well as cutaneous, wound, ocular and catheter-related infections. Image provided by J. Janzen & L. Hall-Stoodley. A scanning electron microscopy (SEM) image of a M. fortuitum biofilm is shown for comparison (right). Single cells (blue arrow), branching cells (red arrow) and putative EPS (green arrow) are indicated. The SEM has higher resolution but requires dehydration which can result in artifacts. The combination of microscopic techniques enhances interpretation of morphological data.

|

Definitions

|

|

|

|

|
What are Biofilms?
Biofilms are difficult to define because of their diversity, both in terms of the micro-organisms that inhabit them and the environmentsin which they are found. One working definition is:
Biofilms are communities of micro-organisms encased within an extracellular polymeric slime (EPS) matrix living on surfaces.
Biofilm microbiology is often contrasted with planktonic microbiology.
What are Planktonic Cells?
Planktonic or free-floating microorganisms come in a number of forms:
Planktonic cultures are cultures that are "forced" to grow primarily as single cells under constant mixing in conventional chemostat or batch shake flask type cultures.
Microbial flocs have many of the same characteristics as biofilms but are not attached to a solid surface. Flocs are suspended aggregates of micro-organisms within an EPS matrix that GREW in liquid suspension.
Detached biofilm particles can be single cells or clumps of cells that GREW on a surface in a biofilm and subsequently detached due to either a biological or environmental stimuli. In an infectious disease context "biofilm emboli" may be a useful term for detached biofilm.
|

|
|
At the CGS we are particularly interested in the dynamic nature of biofilms and how these composite microbial communities change over time, moving, shedding and re-growing adherent colonies.
The spatio-temporal aspect of biofilm development underlies several key research questions that remain to be elucidated such as:
- Why are biofilms less susceptible to antimicrobial treatments than free-floating bacterial cells?
- How do biofilm organisms respond to surfaces genetically and phenotypically?
- How does biofilm development proceed over time?
- How do hydrodynamics and shear affect biofilm development, structure and detachment?
- How does detachment of the biofilm play a role in overall biofilm development?
These questions require state-of-the-art microscopic imaging capabilities in order to examine the spatio-temporal relationships between biofilm structure and function. Both confocal microscopy and real-time imaging are essential components of these investigations that allow us to use fluorescent molecular reporter probes to follow gene regulation during biofilm development
Biofilms and Infection
In industrial systems cells on surfaces (biofilms) may be difficult to culture, hard to kill and persist for long periods of time (years) resulting in problems of chronic contamination. Similar criteria are now increasingly being used to flag a putative biofilm etiology for chronic illnesses in which signs and symptoms suggest infection, but cultures may be negative and the illness does not respond to antibiotic treatment. The Biofilm Paradigm may explain these contravening data. Initial links between biofilms and persistent infection were made in the context of indwelling devices such as catheters and implants. For an online CDC review see Biofilms and Device-Associated Infections by Rodney M. Donlan. More recently the Biofilm Paradigm has been increasingly applied to explain infection on native tissue. These diseases include periodontitis (teeth and gums), tonsillitis (tonsils), Cystic Fibrosis respiratory illness (lungs) otitis media (middle ear), osteomyelitis (bone) and urinary tract infections (bladder).
Research at the Center for Genomic Sciences has revealed that chronic otitis media with effusion (OME), once thought to be a sterile inflammatory process, may in fact be a biofilm disease. Similar combinatorial techniques of confocal microscopy and genotyping are being used to investigate other diseases.
Mucosal biofilm formation on middle-ear mucosa in the chinchilla model of otitis media. Ehrlich GD, Veeh R, Wang X, Costerton JW, Hayes JD, Hu FZ, Daigle BJ, Ehrlich MD, Post JC. JAMA. 2002 Apr 3;287(13):1710-5.
Confocal images of Haemophilus influenza cells and biofilm (green) associated with host cells (nuclei stained red) 24 hours (A) and 10 days (B) post inoculation
Controlling the Growth of Biofilms
An active area of research is in the development of materials and surfaces that resist or prevent biofilm formation. In industry this can mean reduced operating costs and reductions in product spoilage, while in medicine the prevention of biofilms on implants and medical equipment may result in reductions in patient morbidity and mortality.
Pseudomonas aeruginosa PAO1 (pMF230) biofilms grown on untreated polyurethane (left panel) and polyurethane with incorporated usnic acid (right panel) in flow cells. Polyurethane is used to make catheters and usnic acid is a natural "anti-biofilm" antibiotic produced by lichens, a biofilm system composed of fungi, algae and/or cyanobacteria. In Staphylococcus aureus, a Gram positive human pathogen, biofilm formation was inhibited. Intriguingly, in P. aeruginosa biofilm formation was not inhibited but the structure was changed. This is interesting since usnic acid has structural similarities with the homoserine lactones, a family of cell signaling molecules involved in the maturation of biofilm architecture. This work was done in collaboration with Gianfranco Donelli and Iolonda Francolini from University of Rome "La Sapienza". Individual bacterial cells have been genetically engineered to produce GFP for live visualization. The strain was constructed and gifted by Mike Franklin, Dept. Microbiology, MSU-Bozeman.
Francolini, I., Norris,P.M., Piozzi, A., Donelli, G. and Stoodley, P. 2004. Usnic acid as a natural inhibitor of biofilm formation on polymer surfaces. Antimicrob. Agents. Chemother. 48(11): 4360–4365.
Streptococcus pneumoniae, Biofilm Formation and Ear Infection
Streptococcus pneumoniae is a significant human pathogen which can cause a variety of infections. In addition to pneumonia this bacterium can cause sinusitis, otitis media (infection of the middle ear) ear infection), meningitis, osteomyelitis, septic arthritis, endocarditis, peritonitis, pericarditis, cellulitis, and brain abscess. (http://en.wikipedia.org/wiki/Streptococcus_pneumoniae). Recently it has been shown that S. pneumoniae can form biofilms (Allergrucci et al. 2006) and such biofilms are present in the middle ear of children suffering from repeated episodes of otitis media (Hall-Stoodley, et al. 2006). Since biofilms are resistant to many antibiotics we suspect that biofilm formation may explain why infections by S. pneumoniae and other organisms may be so difficult to treat in some people.
This movie shows the dynamic motility of S. pneumoniae (BS72) biofilm "towers" grown in vitro for six days and examined in situ in real time by confocal microscopy. The movie shows sections from top to bottom through the tower. In the movie the detachment of cells and clusters of cells into the surrounding fluid can be seen. Detachment of bacteria from biofilms has been well documented in other types of bacteria and suggests that in the presence of effusion in the ear, individual cells and clusters of pneumococci could readily detach from biofilm aggregates and propagate to other sites. Therefore, biofilm development may also increase the risk of recurrent OM and other acute exacerbations, due to repeated cycles of shedding of pneumococci from biofilm sources in the nasopharynx.
S. pneumoniae Type 23 biofilm with a large mushroom structure protruding from the surface. The extracellular polymeric slime (EPS) matrix was stained green with lectins. Bacteria were stained red with the nucleic acid stain Syto59. Co-localization of these stains appears yellow. Scale = microns.
Model showing progression of pneumococcal biofilm starting with the attachment of cells to a surface, the growth of those bacteria into towers, and the detachment of the tops of the towers which can then reattach elsewhere.
Allegrucci M, Hu FZ, Shen K, Hayes J, Ehrlich GD, Post JC, Sauer K. 2006. Phenotypic characterization of Streptococcus pneumoniae biofilm development. J Bacteriol. 188(7):2325-35.
Hall-Stoodley, L., Hu, F.Z., Gieseke, A., Nistico, L., Nguyen, D., Hayes, J., Forbes, M., Greenberg, D.P., Dice, B., Burrows A., Stoodley, P., Post, J.C., Ehrlich G.D., and Kerschner, J. 2006. Direct detection of bacterial biofilms on the middle-ear mucosa of children with chronic otitis media [Clinical investigation]

Biofilm Links

|

|

|
- Center for Biofilm Engineering
Home page and biofilm movies
- ASM Microbelibrary
Pictures and movies of bacterial cells and biofilms.
- Biofilms Online
Latest in biofilm news and research.
- Biofilm Club
News and updates of biofilm research in the UK and Europe.
- O'Toole Biofilm Lab
Biofilm research from Dartmouth.
|

|
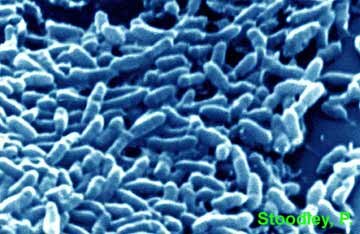
Scanning electron micrograph of a QS BHL mutant Pseudomonas aeruginosa PANO67 biofilm streamer grown under turbulent flow. Image colored by Kathy Lange, CBE, MSU-Bozeman.
|
|